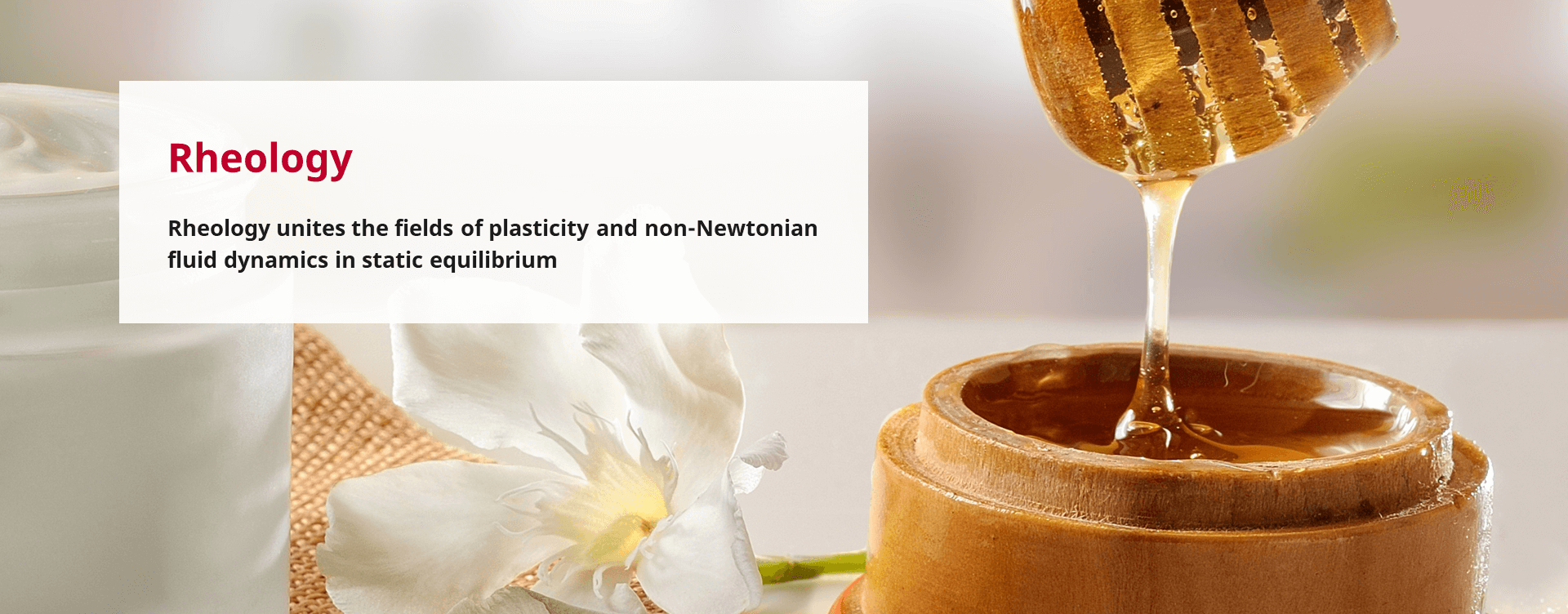
Rheology unites the seemingly unrelated fields of plasticity and non-Newtonian fluid dynamics by recognizing that materials undergoing these types of deformation are unable to support stress (particularly shear stress, since it is easier to analyze shear deformation) in static equilibrium. In this sense, solid undergoing plastic deformation is fluid, although no viscosity coefficient is associated with this flow. Granular rheology refers to the continuum mechanical description of granular materials.
One of the major tasks of rheology is to empirically establish the relationships between strains (or rates of strain) and stresses, by adequate measurements, although a number of theoretical developments are also required before using the empirical data. These experimental techniques are known as rheometry and are concerned with the determination of well-defined rheological material functions. Such relationships are then amenable to mathematical treatment by the established methods of continuum mechanics.
Shear Rheometry
The characterization of flow or deformation originating from a simple shear stress field is called shear rheometry (or shear rheology). The study of extensional flows is called extensional rheology. Shear flows are much easier to study and thus much more experimental data are available for shear flows than for extensional flows.
Rheology has applications in materials science, engineering, mining & minerals, food, life science, and pharmaceuticals. Materials science is utilized in the production of many industrially important substances, such as cement, paint, and chocolate, which have complex flow characteristics. In addition, plasticity theory has been similarly important for the design of metal forming processes. The science of rheology and the characterization of viscoelastic properties in the production and use of polymeric materials has been critical for the production of many products for use in both the industrial and military sectors. The study of flow properties of liquids is important for pharmacists working in the manufacture of several dosage forms, such as simple liquids, ointments, creams, pastes, etc. The flow behavior of liquids under applied stress is of great relevance in the field of pharmacy. Flow properties are used as important quality control tools to maintain the superiority of the product and reduce batch-to-batch variations.
Applications of Rheology
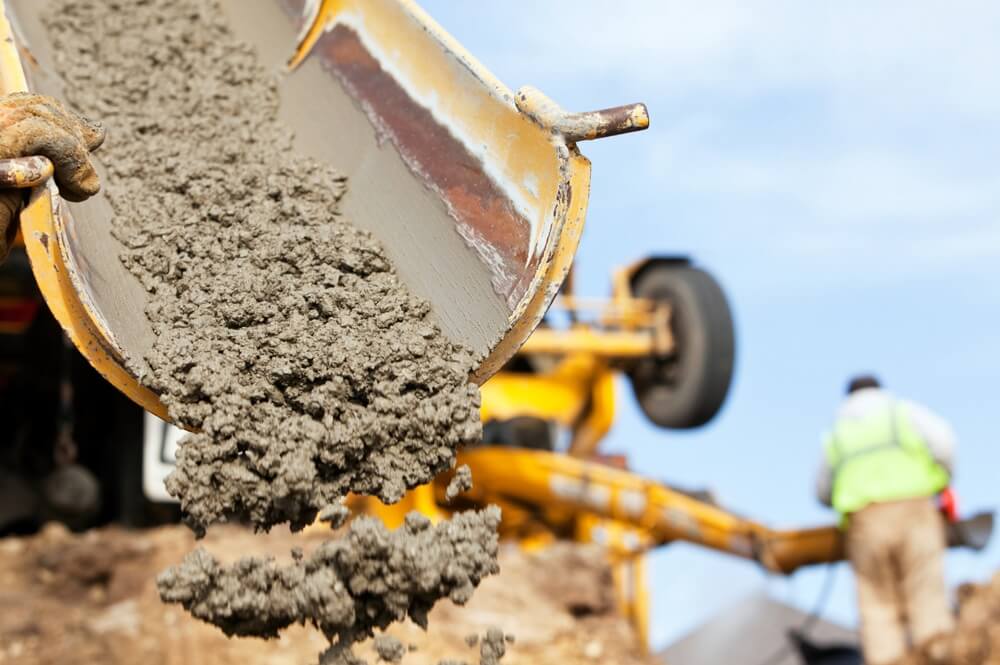
Concrete’s and mortar’s workability is related to the rheological properties of the fresh cement paste. The mechanical properties of hardened concrete increase if less water is used in the concrete mix design, however reducing the water-to-cement ratio may decrease the ease of mixing and application. To avoid these undesired effects, superplasticizers are typically added to decrease the apparent yield stress and the viscosity of the fresh paste. Their addition highly improves concrete and mortar properties.
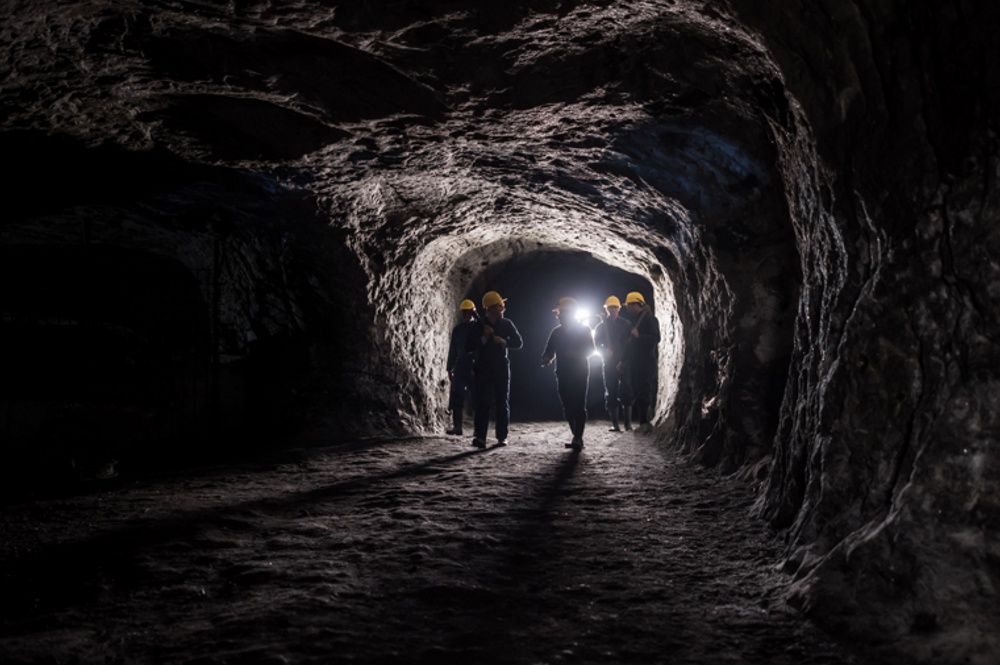
The scientific discipline of geophysics includes the study of the flow of molten lava and the study of debris flows (fluid mudslides). This disciplinary branch also deals with solid Earth materials which only exhibit flow over extended time scales. Those that displays vicious behavior are known as rheids. For example, granite can flow plastically with negligible yield stress at room temperatures (i.e. a viscous flow). Long-term creep experiments (~10 years) indicate that the viscosity of granite and glass under ambient conditions is on the order of 1020 poises.
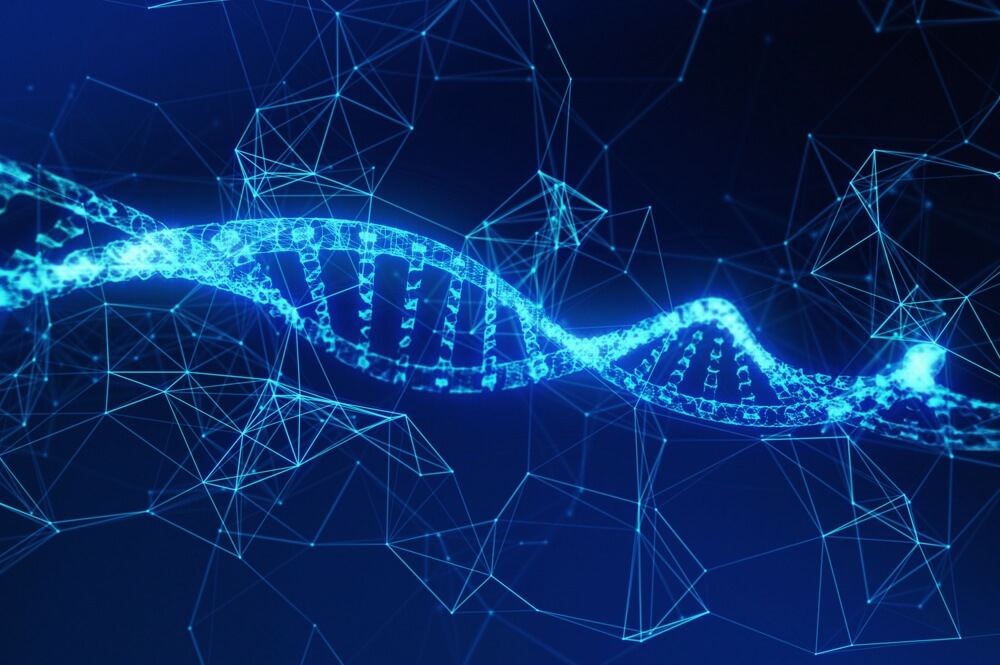
Physiology includes the study of many bodily fluids that have complex structure and composition, and thus exhibit a wide range of viscoelastic flow characteristics. In particular, there is a specialist study of blood flow called hemorheology. This is the study of the flow properties of blood and its elements (plasma and formed elements, including red blood cells, white blood cells, and platelets). Blood viscosity is determined by plasma viscosity, hematocrit (volume fraction of red blood cells, which constitute 99.9% of the cellular elements), and mechanical behavior of red blood cells. Therefore, red blood cell mechanics is the major determinant of the flow properties of blood.
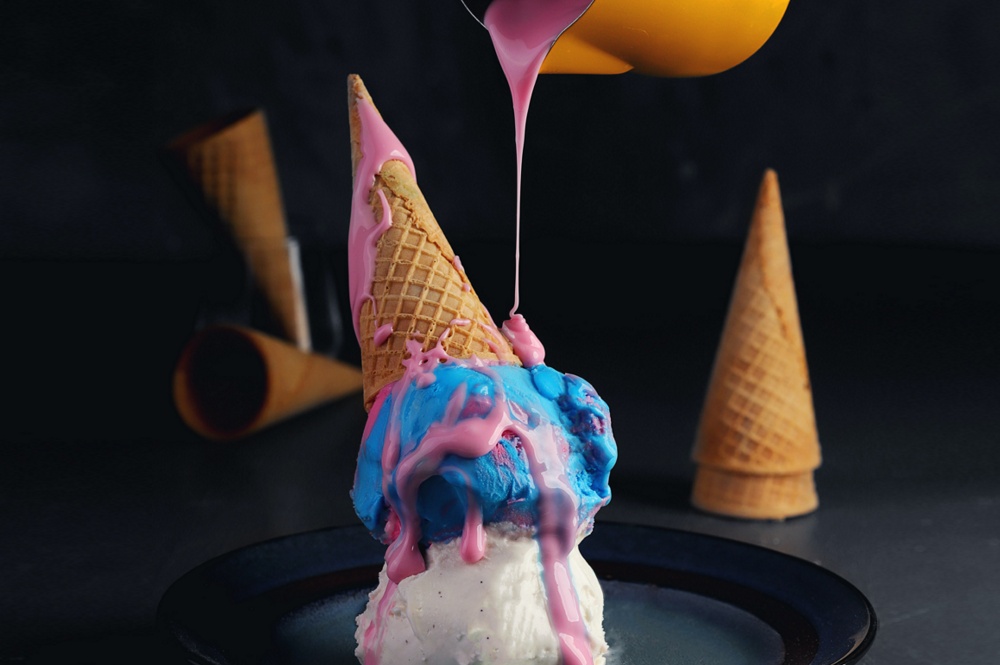
Food rheology is important in the manufacture and processing of food products, such as cheese and gelato.
Thickening agents, or thickeners, are substances that, when added to an aqueous mixture, increase its viscosity without substantially modifying its other properties, such as taste. They provide body, increase stability, and improve the suspension of added ingredients. Thickening agents are often used as food additives and in cosmetics and personal hygiene products. Some thickening agents are gelling agents, forming a gel. The agents are materials used to thicken and stabilize liquid solutions, emulsions, and suspensions. They dissolve in the liquid phase as a colloid mixture that forms a weakly cohesive internal structure. Food thickeners frequently are based on either polysaccharide (starches, vegetable gums, and pectin) or proteins.
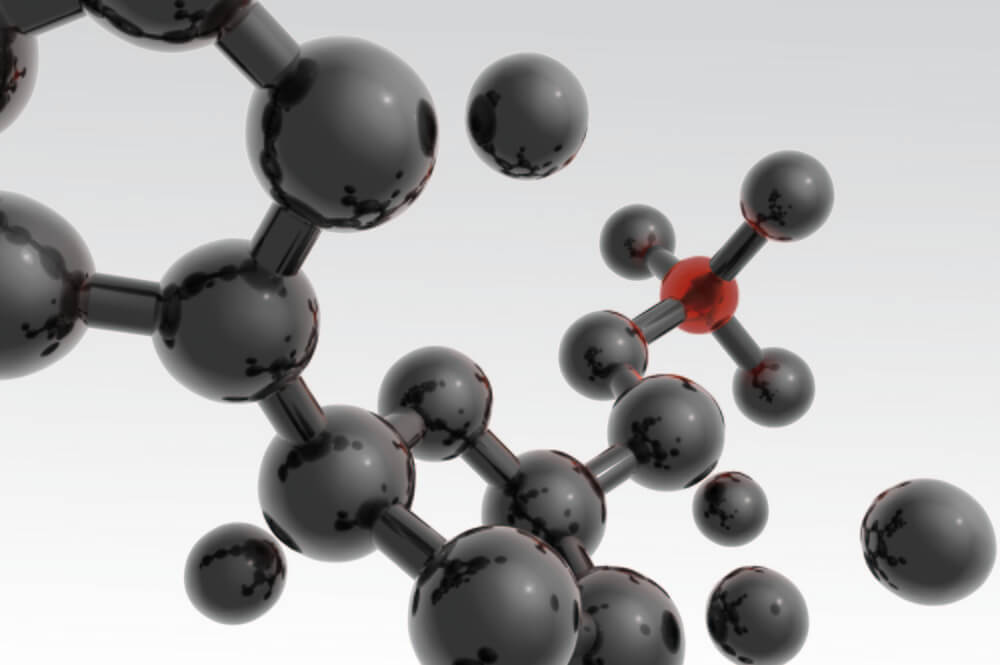
The linear structure of cellulose — the most common component of all organic plant life on Earth. *Note the evidence of hydrogen bonding which increases the viscosity at any temperature and pressure. This is an effect similar to that of polymer crosslinking, but less pronounced.
The polymerization process of tetraethylorthosilicate (TEOS) and water to form amorphous hydrated silica particles (Si-OH) can be monitored rheologically by a number of different methods. With the viscosity of a sol adjusted into a proper range, both optical quality glass fiber and refractory ceramic fiber can be drawn which are used for fiber optic sensors and thermal insulation, respectively. The mechanisms of hydrolysis and condensation, and the rheological factors that bias the structure toward linear or branched structures are the most critical issues of sol-gel science and technology.
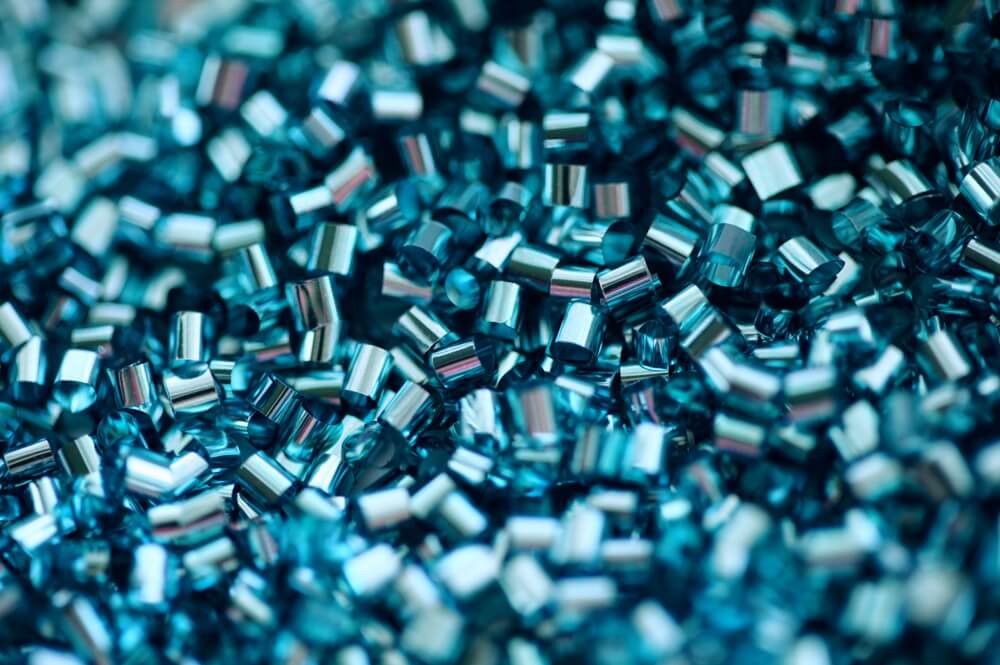
Examples may be given to illustrate the potential applications of these principles to practical problems in the processing and use of rubbers, plastics, and fibers. Polymers constitute the basic materials of the rubber and plastic industries and are of vital importance to the textile, petroleum, automobile, paper, and pharmaceutical industries. Their viscoelastic properties determine the mechanical performance of the final products of these industries, and also the success of processing methods at intermediate stages of production.
In viscoelastic materials, such as most polymers and plastics, the presence of liquid-like behavior depends on the properties and so varies with the rate of applied load, i.e., how quickly a force is applied. The silicone toy ‘Silly Putty’ behaves quite differently depending on the time rate of applying a force. Pull on it slowly and it exhibits continuous flow, similar to that evidenced in a highly viscous liquid. Alternatively, when hit hard and directly, it shatters like silicate glass.
In addition, conventional rubber undergoes a glass transition (often called a rubber-glass transition). E.g. The Space Shuttle Challenger disaster was caused by rubber O-rings that were being used well below their glass transition temperature on an unusually cold Florida morning and thus could not flex adequately to form proper seals between sections of the two solid-fuel rocket boosters.
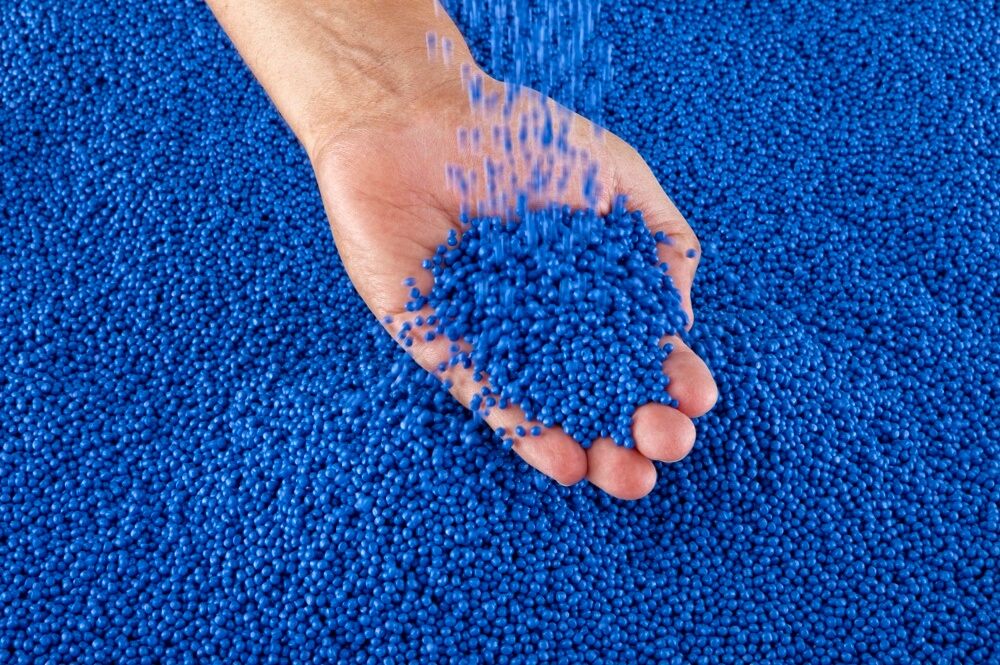
The incorporation of various types of fillers into polymers is a common means of reducing cost and imparting certain desirable mechanical, thermal, electrical, and magnetic properties to the resulting material. The advantages that filled polymer systems have to offer come with increased complexity in the rheological behavior.
Usually, when the use of fillers is considered, a compromise has to be made between the improved mechanical properties in the solid-state on one side and the increased difficulty in melt processing, the problem of achieving uniform dispersion of the filler in the polymer matrix and the economics of the process due to the added step of compounding on the other. The rheological properties of filled polymers are determined not only by the type and amount of filler but also by the shape, size, and size distribution of its particles. The viscosity of filled systems generally increases with increasing filler fraction. This can be partially ameliorated via broad particle size distributions via the Farris effect. An additional factor is the stress transfer at the filler-polymer interface. The interfacial adhesion can be substantially enhanced via a coupling agent that adheres well to both the polymer and the filler particles. The type and amount of surface treatment on the filler are thus additional parameters affecting the rheological and material properties of filled polymeric systems.
It is important to take into consideration wall slip when performing the rheological characterization of highly filled materials, as there can be a large difference between the actual strain and the measured strain.
Related products
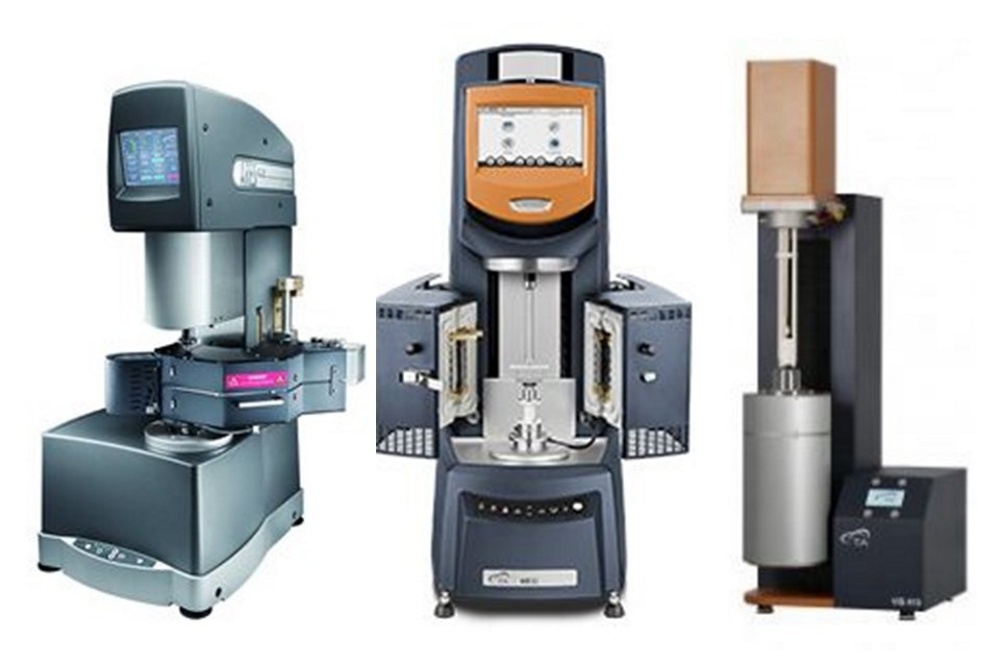
TA instruments Product Range
TA instruments is the world’s leading supplier of thermal analysis, rheology, and microcalorimetry instruments, and our product areas have expanded to include thermal conductivity & diffusivity, dilatometry, rubber testing, and dynamic mechanical characterization.